“Been in America”: Interviews with German researchers in the USA and Canada
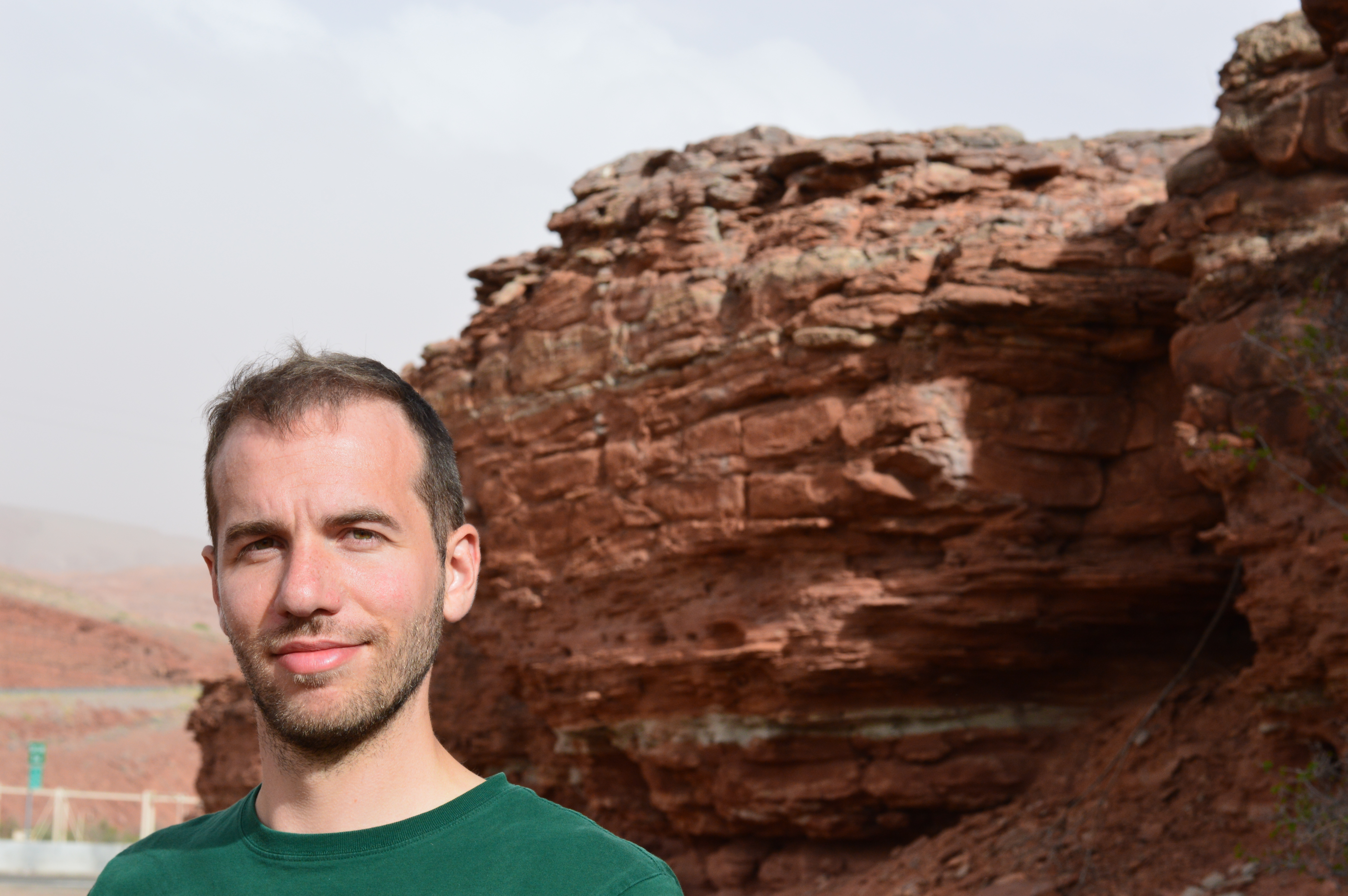
Tim Kodalle
© Tim Kodalle
(07/30/21) Physicist Tim Kodalle is currently at Lawrence Berkeley National Laboratory (LBL) in California on a Walter Benjamin Fellowship provided by the DFG, where he is working on optimizing solar cells in Carolin Sutter-Fella’s group.
His interview with the DFG Office North America covered subjects such as his current research topic, international competition for beam time at a synchrotron, the problems with interfaces and some of the differences between a National Lab in the US and a Helmholtz Association facility.
Through its research fellowship program and the Walter Benjamin Program, the DFG supports junior scientists in their academic careers by funding an independent research project abroad and, since 2019, also in Germany. A large proportion of these fellowships are awarded in the USA, and to a lesser extent in Canada, thereby reflecting the belief still prevalent in many disciplines that for a career in research it is helpful to have “been in America.” In this series of talks, we aim to give you an impression of the wide range of DFG funding recipients. In this edition we take a look at who is behind funding number KO 6414.
DFG: Dr. Kodalle, many thanks for taking the time to talk to us. You are an experimental physicist currently at Lawrence Berkeley National Laboratory in California. Is this a childhood dream come true for you -- did you always want to become an experimental physicist?
Tim Kodalle (TK): It might seem straightforward from today’s perspective but in fact things were a little more circuitous than that, and chance was an important factor – if not the most important. But first let me say how grateful I am to the DFG for giving me the opportunity to conduct research here in California on ways to optimize solar cells. As far as my career is concerned: I was born not far from the DFG headquarters (on the other side of the Rhine, in Troisdorf) but I grew up near Bremen. That was where my father had ended up; he worked as a sales representative for a manufacturer of doors and windows and was assigned to a new area. My mother is a trained office and industrial salesperson and I have three siblings who have become a carpenter, a nurse and an engineer – so they seem to have inherited our parent’s practical skills. I’ve always been more concerned with theoretical matters and was the only one to delve deeper into research.
DFG: At what point did you make the decision to go into physics and research?
TK: It was a combination of a few key factors you’re probably already familiar with: Very good teachers in the relevant subjects at school, my personal inclinations at upper secondary school, an understanding of mathematics that was probably above average, and finally, simply a series of coincidences, or shall we say opportunities that arose by chance (the word “serendipity” captures this rather well). I did my civilian national service in the Department of Nuclear Medicine at the Münster University Hospital. The excellent technical staff there – mostly engineers and physicists -- motivated me to move into the technical direction as well. Therefore, it was just a small step from there to the physics department at the University of Münster. During my bachelor’s degree and subsequent master’s degree studies, I continued to work in nuclear medicine clinics, so you might even say I pursued something like a dual training course. As such, my interest in physics developed from a practical perspective. I have nothing against the question of “why,” but I personally tend more towards the question of “how” – perhaps because solutions are beginning to emerge, even if this often only happens in frustratingly small steps.
DFG: But as your career has progressed to date, the steps you’ve made have been somewhat bigger and not as frustrating, haven’t they?
TK: Certainly not frustrating – whether they’ve been really big steps remains to be seen. After completing my bachelor’s thesis in Münster, I moved to Berlin in 2013 to study for a master’s degree at Humboldt University, which is when I started to specialize in my current field. While my bachelor’s thesis was about a correction mechanism for mirrors in the European XFEL in Hamburg, i.e. an X-ray laser, my master’s thesis dealt with methods for producing very thin semiconductor films for solar cells. I then went on to the Helmholtz Center for Materials and Energy in Berlin (HZB) to pursue my doctoral studies, and my alma mater was the University of Halle-Wittenberg (MLU). There I received my doctorate under Roland Scheer and Rutger Schlatmann with a thesis on the use of rubidium in solar cells. Professor Scheer heads a large and very successful photovoltaics group in Halle, while Professor Schlatmann is at the HZB as well as the Berlin University of Applied Sciences (HTW), where he holds a chair in environmental technology and renewable energies. Both are among the global avant-garde when it comes to the further development of solar cells and I consider myself very lucky to have been supported by them. For me, the attraction of combining studies at MLU and HZB lay especially in being able to conduct basic research into the structural and optoelectronic effects of rubidium in chalcopyrite-based solar cells as well as being involved in the transfer of these findings to industry. This might be a reflection of the family urge to be close to practical application.
DFG: That doesn’t sound like a broad career plan with the ultimate goal of a professorship.
TK: It’s not something I would ever rule out, but as things currently stand, I wouldn’t apply to an early-career research program such as Emmy Noether. My research profile is actually not broad enough for that and my research interest is too close to industrial applications. This doesn’t mean I don’t have the ambition to lead a research group, but it would have to be at a non-university institution or even in industry. My main concern at the moment is to move physics ahead with a view to meeting technological needs, thereby helping to get a better grip on the serious problems of our times such as climate change and renewable energies.
DFG: What exactly are these problems at the moment?
TK: The sun is capable of giving us more than enough energy here on Earth if only we were able to put this energy to more efficient use to meet our needs. In the field of hydroelectric power, this already works very well: the sun evaporates water, it rains, we collect the water, the collected water runs down the mountain, we put a turbine in the flow of water and this generates electricity. With wind it is similar, perhaps a little more complicated – and I wouldn’t want to offend anyone here with a gross simplification. If you want to convert sunlight directly into electricity, you need a material that can absorb the energy of sunlight and convert it with maximum efficiency into what we want – namely electrical energy. When you buy a solar module today, it might manage just under 20%. In the lab, under better conditions, we can get an even higher rate. Considering the fact that the theoretical maximum, i.e., the best possible efficiency of a “simple” solar cell is about 33.7%, this is not bad at all.
DFG: What causes this limitation?
TK: Semiconductors are used to absorb sunlight, and their absorption spectrum is determined by their so-called energy band gap. The semiconductors we are looking into absorb higher-energy light almost completely, but also leave unused much of the incident light, which is lower-energy than this band gap. Additionally, there are some unavoidable losses due to thermalization effects. It’s possible to optimize the band gap based on a clever choice of materials, but only up to the 33.7% efficiency rate I mentioned before. This is because very small band gaps lead to very good absorption and therefore high currents, but unfortunately the voltage of the solar cell inevitably suffers. The obvious idea is then to combine materials with different band gaps, i.e. to develop simple component structures into double-layered ones. Using so-called tandem solar cells, you can achieve a theoretical efficiency of 42%, i.e. more than double what the solar module on the roof of your house currently produces.
DFG: Impressive! So why not fan the sunlight out even further and have it absorbed by more than just two layers?
TK: This is already being done at the laboratory level – and quite successfully, at that. But the devil is not only in the detail: the main problem are the interfaces between the individual layers. There is an apt quote on this by the physicist and Nobel Prize winner Wolfgang Pauli: “The volume of the solid was created by God, but its surface was made by the devil.” A “simple” solar cell already consists of several layers – in my case five – and the respective interfaces. A lot of things come together in multi-layer solar cells – you have no idea what kind of problems you can get into. Even if we could come to grips with these problems, the increase in theoretically potential efficiency would no longer be in the same range as it is in the step from single-layer to double-layer solar cells. You have to consider cost efficiency too, of course. So in my opinion, the step towards tandem solar cells is the most promising in terms of achieving cheaper, mass-produced solar cells with an efficiency of perhaps 30%. That would be a huge leap forward. As a comparison, the combustion engines under the hoods of our cars don’t achieve 30% efficiency in normal operation, yet we’ve been using them for more than 100 years. The leap to 30% efficiency in solar cells is currently underway and we need to achieve it in order to quickly meet energy needs in a more sustainable way. But I would like to emphasize once again that the solar modules available on the market today are already efficient enough to generate electricity in many regions of the world at least as cheaply as fossil fuels can. Nonetheless, with an even higher degree of efficiency we could contribute to making solar energy even more attractive so as to be able to “force” it even more emphatically on policymakers, business, and industry.
DFG: Who is “we”? How many people are currently working on optimizing solar cells and how many are there in your specialist field?
TK: In terms of photovoltaics research and development, there are probably several thousand worldwide; in my specific field, I would imagine there are a few hundred researchers. That depends a little on how broadly you define my current research topic.
DFG: In your project proposal, you describe work on perovskites, whose crystal growth you aim to understand and control more effectively so as to make two-layer solar cells more efficient. How would you explain this to a group of non-physicists?
TK: Exactly. For some years now, the photovoltaic world has been working very intensively on a new, highly efficient semiconductor material – called perovskite. Perovskites have unique properties, including the ability to adjust the above-mentioned energy band gap based on the ratios of the elements contained in the material. This makes them ideal as a second solar cell in a tandem, since they can be optimally adjusted to the partner (a conventional silicon solar cell, for example). The functional principle of such silicon perovskite tandems has already been demonstrated very impressively by colleagues at HZB, who recently presented a record cell with 29% efficiency. But that still leaves a lot of potential for achieving the tandem limit of 42%. And to do that, we need to better understand how the surface of the lower solar cell affects the crystal growth of the perovskite. This is where the strengths of my new group here in Berkeley come into play. For quite some time, Carolin Sutter-Fella’s group has been working on the development of methods for in-situ characterization of materials, i.e. characterization during crystal growth. By doing this, we can examine very precisely the development of the absorption spectra of the perovskite over time, for instance, gaining a very accurate understanding with a time resolution in the range of 0.001 seconds.
DFG: What does a project like this involve in terms of day-to-day activity?
TK: I spend most of my time in the lab preparing experiments for the brief moments when I get measuring time on the large-scale equipment. There are various methods for the production of metal halidess and techniques for the characterization and determination of defects and impurities. I have a good collection of smaller instruments and devices at my disposal, some of which have been developed by the group; I also have colleagues with a lot of experience in using the equipment and I can engage with my group and my group leader. The apparent chaos in experimental physics laboratories is deceptive: we actually proceed in a very systematic and orderly manner, otherwise we wouldn’t get any measuring time on the big machines.
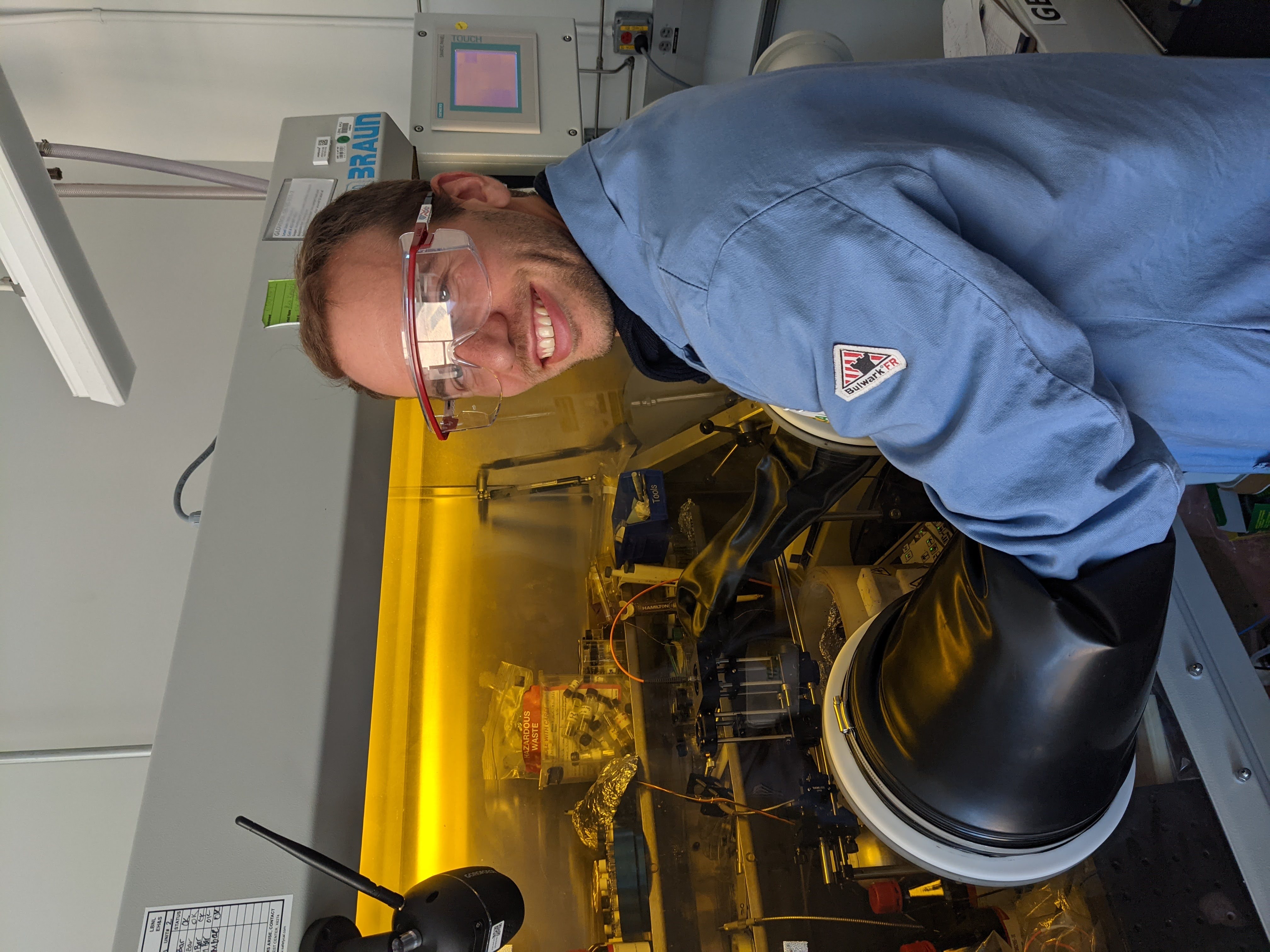
Preparations for beam time
© Tim Kodalle
DFG: In past years you were at HZB with the keys to BESSY, the big machine in Berlin, and now you’re at Lawrence Berkeley National Laboratory. Didn’t they give you a key to the Advanced Light Source (ALS) – the big machine there?
TK: That would be nice for me, but probably not a good thing for science as a whole. No matter how close you are to a synchrotron, i.e. in Berlin near BESSY or now here in Berkeley directly at the ALS, you have to apply for measuring times at these large machines and you only enjoy a “home advantage” to a limited extent. These are very expensive and therefore rare facilities that are financed by the general public. There are many other important topics besides solar research of course, so the periods of use are put out to tender worldwide and awarded based on an orderly peer-review process. So you can consider yourself lucky if you’re able to get “beam time”, i.e., an appointment at one of the synchrotron’s few measuring stations. If you do get a place, it’s a matter of “24/7,” that is you have to use it around the clock. We do that as a team.
DFG: What is the approval rate for “beam time”?
TK: This again depends very much on the exact requirements of the experiment – each synchrotron has numerous different measuring stations for a wide variety of applications, so demand varies significantly. In my case at the ALS, I think the approval rate is marginally higher than the success rate of football goalkeepers in penalty shoot-outs – but probably with fewer surprises.
DFG: If measurement times are so coveted, how are the measurement results handled?
TK: That’s an interesting question. First of all, the measurement results serve as a basis for academic publications produced by those who have done the measurements. However, other insights could certainly be gained from the data that might not have been the focus of those doing the measuring. So journals increasingly want to see all the measurement data published, partly for the sake of reproducibility and transparency, but presumably also because it may be possible to derive findings other than those just published.
DFG: This is the purpose of the National Research Data Infrastructure (NFDI), an organization founded last October as an independent association for the more efficient use of research data; the DFG is organizing the calls for proposals for this.
TK: Ah, you see, I’m preaching to the converted, though I do think that a research data infrastructure should be international.
DFG: We do too, but we’re a national research funding organization, even though – as in your case, for example – we also operate internationally, providing international funding and facilitating international collaboration.
TK: Yes, it obviously makes sense to support international collaboration since the majority of projects in science are carried out by international teams, even though team members are nationally sponsored. My collaboration partners outside the US are in Switzerland, Japan and the Netherlands. Moreover, I can’t be grateful enough for my own funding for the Walter Benjamin Fellowship since it gives me a level of freedom here in California that I am occasionally envied for – especially among colleagues whose funding agencies prefer to keep them on a short leash. I’m familiar with the short leash myself from my work on projects funded by the Ministry of Economic Affairs. Sometimes you have them breathing down your neck wanting to see results, so I’m very happy with my DFG funding in California at the moment.
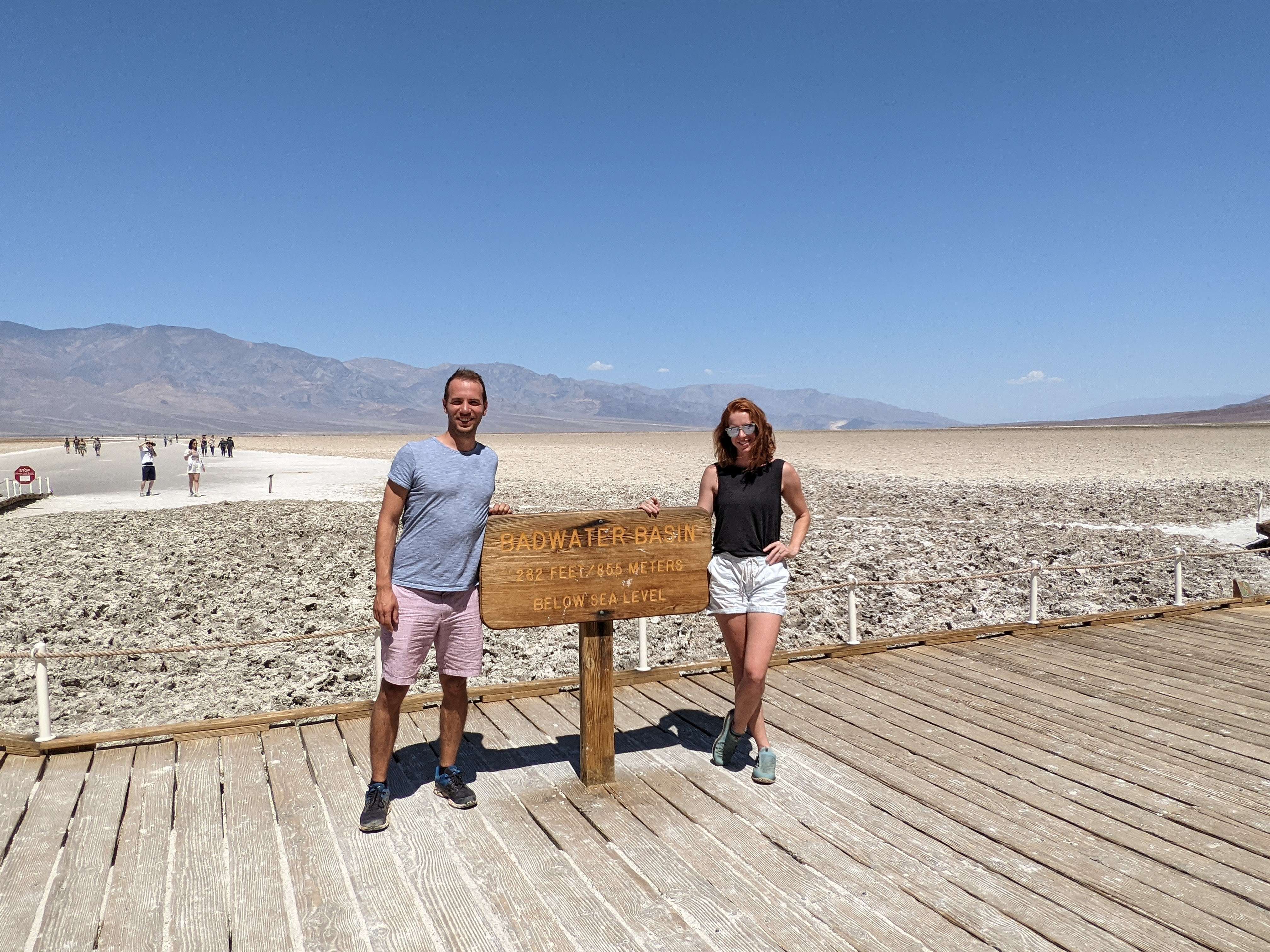
In a very sunny place
© Tim Kodalle
DFG: How much does the Californian landscape contribute to your current state of happiness?
TK: It accounts for a lot because the area here is one of the few regions in the US that I've been able to agree on with my American fiancée as a temporary place to live. And objectively speaking it's of course very appealing to be able to travel back and forth between palm beaches, deserts and high mountains within a few of hours by car and be able to fully enjoy this natural beauty. That’s something that even excites experimental physicists like myself!
DFG: Is your fiancée an experimental physicist, too?
TK: No, she pursues her curiosity in a more artistic way, that is to say not so much inter-subjective as aesthetic – she’s a dancer and yoga instructor. I met her more or less by chance in autumn 2018 during a research stay at the University of South Florida in Tampa. She’s able to appreciate my curiosities just as I can understand hers – but after almost three years of a long-distance relationship, we now want to be in the same place.
DFG: You’re in Berkeley in the group under Carolin Sutter-Fella, whose surname before the hyphen has a particular ring in some parts of California because of John Augustus Sutter.
TK: Well, in San Francisco, Sutter Street is perhaps one of the top addresses, in Sacramento there is even a Sutter Triangle in a prime location, and Sutter Street in Berkeley is a desirable place to live, too. But even in Oakland people are unsure how to spell the name of the rather unfortunate “founding father” of modern California. I haven’t discussed the subject with Carolin yet. She adopted this part of her surname through her husband, who is Swiss, but unlike John Augustus Sutter, she herself is not a citizen of either Switzerland or Mexico. There is one thing they might have in common though: none of John Augustus Sutter's contemporaries in California were US citizens before 1850, because California was not admitted to the Union until 1850. Interestingly, there are no US citizens in Carolin’s group either at the moment.
DFG: Another question in terms of differences and similarities -- you were in Berlin at a facility belonging to the Helmholtz Association, the German equivalent of a US National Lab. Is the equipment at this type of facility comparable? What are the differences?
TK: The facilities are excellent on both sides of the Atlantic. Perhaps Americans are more inclined to ask questions in such a way that money is an answer and more money is an even better answer. This might be reflected in absolute budget figures, but it isn’t so noticeable at the level of the actual work. In the US, I noticed that most of the research work is done by postdocs – researchers who have completed their doctorate – whereas in Germany there were significantly more doctoral students in the team. In addition, the composition of the team in the US is perhaps a little more international – with all the experience, stories, and methods that this involves. Another difference, which mainly concerns my experiments, can be seen in the staffing of the laboratories. In the group under my former boss in Berlin, Christian Kaufmann, we were able to rely on the support of two permanently employed engineers and a technician. You rarely, if ever, find anything like that in the US. There’s a supporting personnel infrastructure at the laboratories in the US, of course, though in my case at LBL the assistants are mainly recruited from the academic sector – and usually do not have long-term contracts. At Berkeley, this includes the building manager, who holds a PhD in chemistry and has himself worked as a researcher in the US, the UK and Germany. This is an advantage because he can sometimes understand the background of my concerns better, but there are occasional disadvantages, too, when it comes to the more or less professional implementation of technical solutions. The support provided by my technical colleagues in Germany was a bit more “straightforward.” But this comparison only reflects a very small section of the academic landscape on both sides.
DFG: What are your plans for the near future?
TK: For the next 20 months at least we will be here in Berkeley, where I hope to get enough beam time to successfully complete my research project and publish some good results. Then we’ll wait and see. My future wife would like to enjoy a lot of the same sunlight which I am trying to enable more efficient use of in developing solar cells. So we might stay longer in California or go back to Florida or some other place with 3,000 hours of sunshine a year. But she is also preparing to make 50% more efficient use of sunlight herself: then we could get by with 2,000 hours of sunshine a year, in which case Berlin would be a really good option for both of us. At the moment we’re not giving any serious thought to this though – we’re simply enjoying the luxury of being in such a beautiful place. I personally also enjoy the luxury of being able to work in such an interesting and productive group.
DFG: In that case we wish you all the best with your applications for beam time, your research project and your contribution to more efficient solar cells, and finally – in the interests of research in Germany – we hope you are successful in your efforts to make your future wife happy with a little less sunlight than in California or Florida.
Sutter-Fella Lab website: